The second… is defined by taking the… transition frequency of the caesium-133 atom, to be 9192631770 when expressed in the unit Hz, which is equal to s⁻¹ ."
06 December 2024
COMMENT: Why timekeeping is now on the verge of a giant leap forward in accuracy
Time is vital to the functioning of our everyday lives: from the watches on our wrists to the GPS systems in our phones.
Communication systems, power grids, and financial transactions all rely on precision timing. Seconds are the vital units of measurement in timekeeping.
Surprisingly, there is still debate over the definition of the second. But recent advances in the world’s most accurate forms of timekeeping may have just changed the game.
Accurate timekeeping has always been part of humankind’s social evolution. At the Neolithic monument of Newgrange in Ireland, a special opening above an entrance allows sunlight to illuminate the passage and chamber on the shortest days of the year, around December 21st, the winter solstice.
Some 2,300 years ago, Aristotle said that “the revolution of the outermost sphere of the heavens” should be the reference for measuring time. The Greek philosopher believed the cosmos was arranged into concentric spheres, with Earth at the centre.
Water clocks, which appeared around 2,000BC, are among the oldest instruments for measuring time. They do this by regulating the flow of water into or out of a vessel. The mechanical clock was then established in the late 13th century.
Up until 1967, a second was defined as 1/86,400 of a day, with twenty-four hours in a day, sixty minutes in an hour and 60 seconds in a minute (24 x 60 x 60 = 86,400). The International System of Units then changed things, settling for this definition:
If you’re confused, let me elaborate. The core of this definition is something called transition frequency. A transition happens when electrons in an atom absorb energy and move to a higher energy level, returning to a relaxed state after time. It’s a bit like drinking a cup of coffee: you suddenly have more energy, until the caffeine wears off. The frequency is the expected number of times a transition occurs for a specific time period.
In every tick of the second, a specific transition of an electron of caesium-133 occurs 9192631770 times. This has become the yardstick of measuring time. To date, caesium provides the most accurate definition of the second, but it can be improved using higher frequencies.
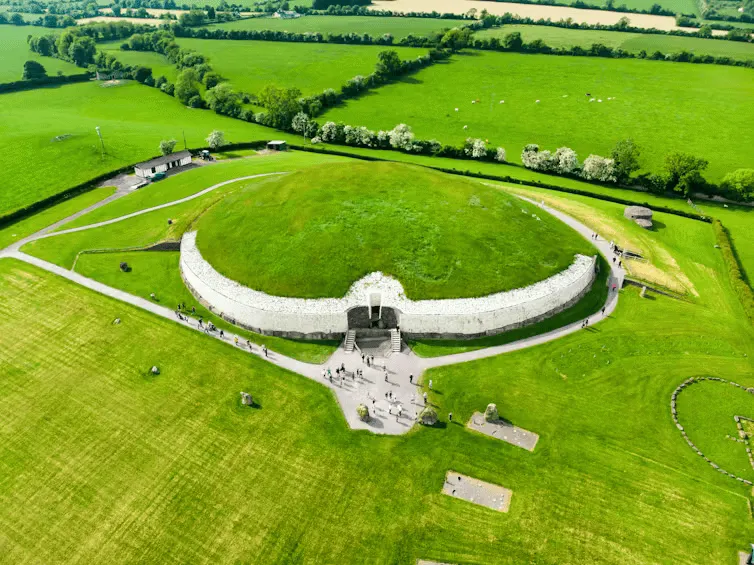
The higher the transition frequency, the less a single misreading can derail the total accuracy. If there were fifty transitions per second, the cost in terms of the accuracy of miscounting one would be one hundred times more severe than if there were 5,000.
There are two limitations in reducing this error: the technological challenges of measuring frequencies, especially higher ones; and the need to find a system – caesium-133 atoms for the second – with a measurable high-frequency transition.
In order to measure an unknown frequency, scientists take a signal of known frequency – a reference – and combine it with the frequency they want to measure. The difference between them will be a new signal with a small frequency that’s easy to measure: the beating frequency.
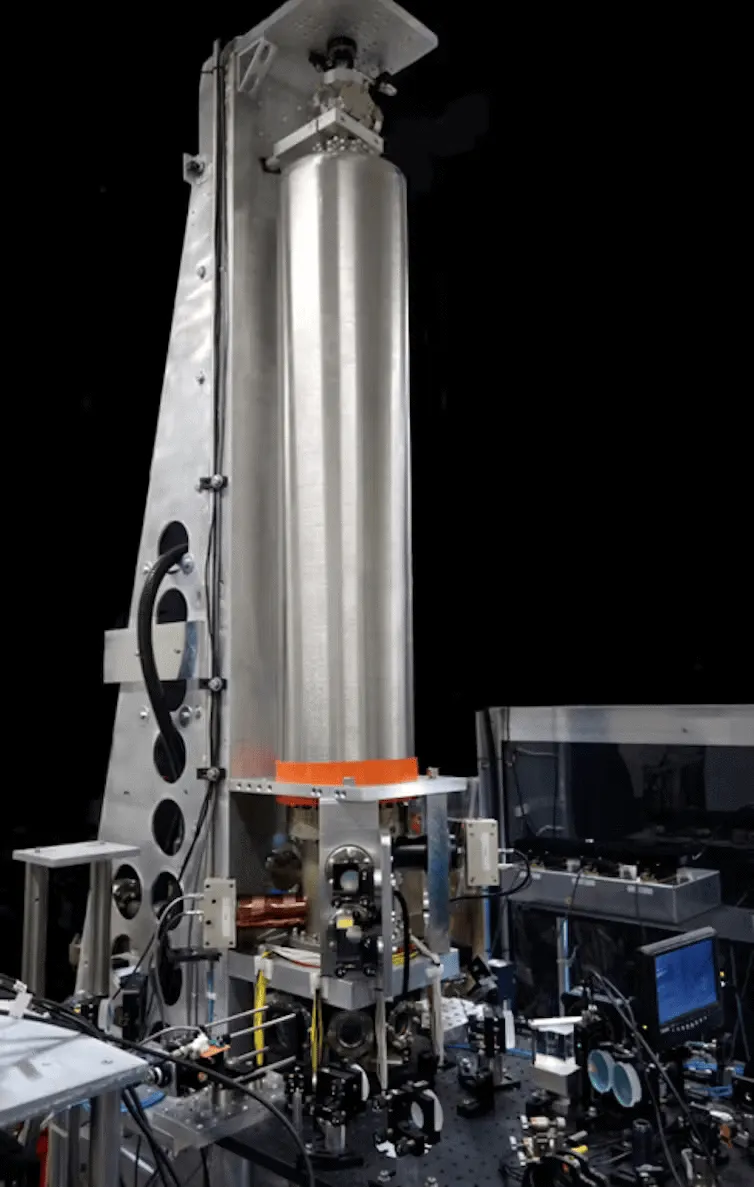
Nist-F4, a caesium fountain atomic clock at the US National Institutes of Standards and Technology. A. Novick/NIST
Atomic clocks use this technique to measure the transition frequency of atoms so precisely that they become standards for defining the second. To achieve such precision, scientists need a reliable reference signal, that they obtain with something called a frequency comb.
A frequency comb uses lasers, beamed out in intermittent pulses. These beams contain many different waves of light, whose frequencies are equally spaced out, like the teeth of a comb – hence the name.
In atomic clocks, a frequency comb is used to transfer energy to millions of atoms simultaneously, hoping for one of the comb’s teeth to beat with the transition frequency of an atom. A frequency comb whose teeth are numerous, thin and in the right range of frequencies increases the chances this will happen. They are therefore key to achieving high precision measurements of a reference signal.
From atomic to nuclear clocks
As we’ve seen, the second is defined by electron transitions in caesium atoms. Transitions occurring with a lower frequency are easier to measure. But those that occur at a higher frequency help increase the accuracy of the measurement.
Caesium transitions occur at around the same frequency on the electromagnetic spectrum as microwaves. These microwave frequencies are lower than those of visible light. But in September 2021, scientists made measurements using the element strontium, whose transition frequency is higher than caesium and falls within the range of visible light. This opens up the possibility of re-defining the second by 2030.
In September 2024, US scientists made key advances towards building a nuclear clock – a step beyond an atomic clock. In contrast to the atomic clock, the transition measured by this new device happens in the nucleus, or core, of the atom (hence the name), which gives it an even higher frequency.
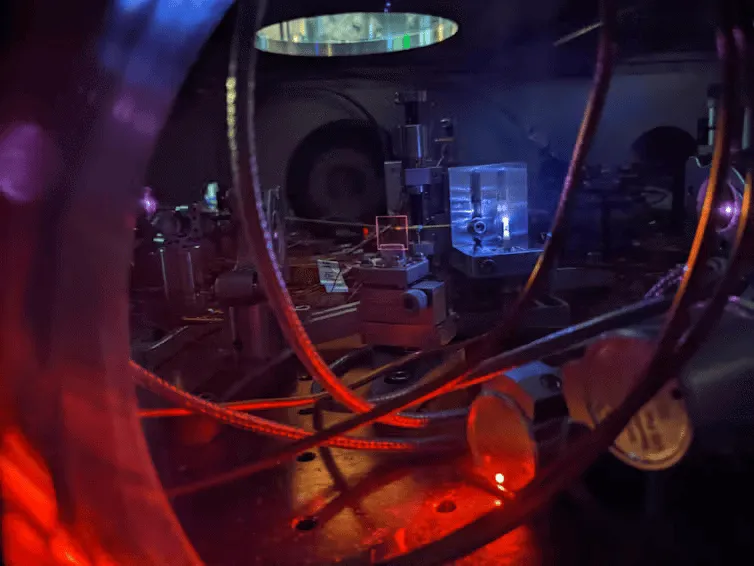
Thorium-229, the atom used for this study, offers a nuclear transition that can be excited by ultraviolet light. The team working on the nuclear clock overcame the technological challenge of building a frequency comb that works at the relatively high frequency range of ultraviolet light.
This was a big step forward because nuclear transitions usually only become visible at much higher frequencies – like those of gamma radiation. But we are not able to accurately measure transitions in the gamma range yet.
The thorium atom transition has a frequency roughly one million times higher than the caesium atom’s. This means that, although it has been measured with a lower accuracy than the current state-of-the-art strontium clock, it promises a new generation of clocks with much more precise definitions of the second.
Measuring time to the nineteenth decimal place, as nuclear clocks could do, would allow scientists to study very fast processes. Think of two runners tied in a photo finish. If the referee’s stopwatch had a few extra digits, they would be able to identify the winner.
Similarly, general relativity is used to study high speed processes that could lead to overlaps with quantum mechanics. A nuclear clock will give us the technology necessary for proving these theories.
On a technological level, precise positioning systems such as GPS are based on complex calculations that require fine measurements of the time required by a signal to jump from one device to a satellite and onto another device.
A better definition of the second will translate to much more accurate GPS. Time might be up for the caesium second, but a whole new world awaits beyond it.
This article is republished from The Conversation under a Creative Commons license. Read the original article