We’ve shown here that it’s a two-way street: taking in a gene might confer an evolutionary advantage, allowing a species like yeast to thrive in specific environments, but it also puts pressure on the host to evolve. It’s a trade-off between having benefits over your competitors and the cost of changing your biology.
Professor Snezhana Oliferenko, Professor in Evolutionary Cell Biology in the Randall Centre for Cell & Molecular Biophysics at King’s
16 April 2025
Beneficial bacterial gene rewires yeast biology
New research has shown that the transfer of genes from bacteria to more complex organisms like yeast can give the new host a survival advantage – but it requires extensive rewiring of the host’s biology.
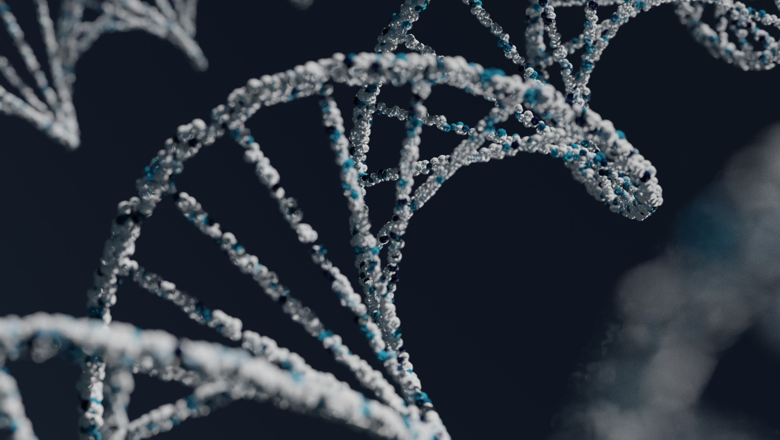
Genes are usually passed down from one generation to the next in what is known as a vertical line of descent. But genes can also be transferred ‘horizontally’ between unrelated organisms. This type of genetic exchange occurs frequently in bacteria and is an important driver of bacterial evolution, allowing the spread of traits like antibiotic resistance between unrelated pathogens.
In a new study from King’s College London and The Francis Crick Institute, and in collaboration with the Hungarian Academy of Sciences, researchers looked at the integration of a horizontally transferred gene that codes for an enzyme called squalene-hopene cyclase (Shc1) from bacteria into a type of yeast called S. japonicus.
The team found that the horizontally acquired gene helps the yeast to survive without oxygen, but it requires significant rewiring of the yeast’s biology. The findings were published in Nature Communications.
“When we think about genes transferring between species, we think about how the gene’s function might change in a new organism. But we don’t always consider how the organism has to adapt to allow the new gene to work,” says Professor Snezhana Oliferenko, Professor in Evolutionary Cell Biology in the Randall Centre for Cell & Molecular Biophysics at King’s and senior author of the paper.
Most species of yeast need oxygen to survive because several biochemical reactions require it. This includes the production of molecules called sterols (ergosterol in yeast and cholesterol in humans), which are needed for functioning cell membranes.
But S. japonicus can survive without oxygen, which is believed to be linked to the transferred Shc1 enzyme. This enzyme generates molecules called hopanoids – that have a similar function to sterols – in the absence of oxygen.
Through a series of experiments in the lab, the team showed that S. japonicus can switch between using an enzyme that generates sterols in the presence of oxygen, called Erg1, and the horizontally acquired Shc1 enzyme to produce hopanoids in conditions without oxygen.
The team then integrated Shc1 into a closely related yeast species, S. pombe, which can’t grow without oxygen and depends on sterols. When S. pombe had Shc1, some hopanoids were produced, but it still relied primarily on sterols.
When the team reduced the production of Erg1 in S. pombe, more hopanoids were produced, suggesting that the enzymes compete for ingredients needed to make both sterols and hopanoids.
As S. japonicus can grow in higher temperatures, the researchers also tested if the addition of Shc1 would allow S. pombe to survive in higher temperatures. With Shc1, S. pombe could survive in higher temperatures and without oxygen, showing that the acquisition of the new gene immediately allowed it to adapt to different environments.
Accommodating new functions
The team then created cell membrane models to look at how yeast could accommodate hopanoids into their cell membranes.
S. pombe has classic cell membranes, formed of two layers of molecules called lipids that have two long fatty acid tails of the same length. But most S. japonicus membrane lipids have fatty acid tails of two different lengths. Studying their cell membrane models using cryo-electron microscopy, the team saw that membranes made from the S. japonicus-like lipids were asymmetrical and much thinner than others.
They found that hopanoids could fit into the membrane better when the fatty acid tails were of different lengths, suggesting that S. japonicus has adapted its lipid metabolism to produce two different lengths of fatty acids to accommodate the hopanoids.
The team concluded that taking in the gene from bacteria provided S. japonicus with an advantage against other yeast species, especially in high temperature and low oxygen environments – however, this survival advantage comes at the cost of rewiring an important part of its biology.
Our work shows that host metabolism must be rewired to accommodate a new metabolic functionality encoded by a horizontally transferred gene. Such changes can, in turn, have systems-level knock-on effects, with profound implications for cellular biology and organismal physiology.
Professor Oliferenko
Several aspects of S. japonicus biology, including cell division and how it controls its shape, are profoundly different from other related yeast species, and the team is now investigating whether the differences in lipid metabolism could lead to changes in complex cellular processes.
The research was led by co-first authors Dr Bhagyashree Rao and Dr Elisa Gomez-Gil, both postdoctoral researchers in the Oliferenko lab. The team collaborated with Dr Qu Chen, Dr Peter Rosenthal, Dr Vanessa Nunes and Dr James Macrae at The Francis Crick Institute, and Dr Maria Peter and Dr Gabor Balogh at the Hungarian Academy of Sciences.
The work was supported by the Wellcome Trust.