What are biomarkers?
The Oxford English Dictionary defines a ‘biomarker’ as:
“A naturally occurring molecule, gene, or characteristic by which a particular pathological or physiological process or disease can be identified.”
In the context of neurological disease, biomarkers can involve tests on bodily fluids (blood, urine, cerebrospinal fluid), brain or spinal cord imaging (e.g. MRI), or electrical tests. Each biomarker needs to undergo sufficient validation, assessing its safety, reliability, reproducibility, cost-effectiveness, ease of use, rapidity and accuracy.
How can biomarkers help?
Biomarkers of disease can play several key roles and it is important to design each study carefully in order to ensure appropriate interpretation. Biomarkers can help with:
- Diagnosis – allowing patients to receive the correct diagnosis earlier in the disease
- Prognosis – allowing patients and doctors to plan for the future
- Disease monitoring – providing the quantitative means to test the efficacy of a novel drug
- Patient stratification – ensuring patients with similar features are grouped appropriately in clinical drug trials
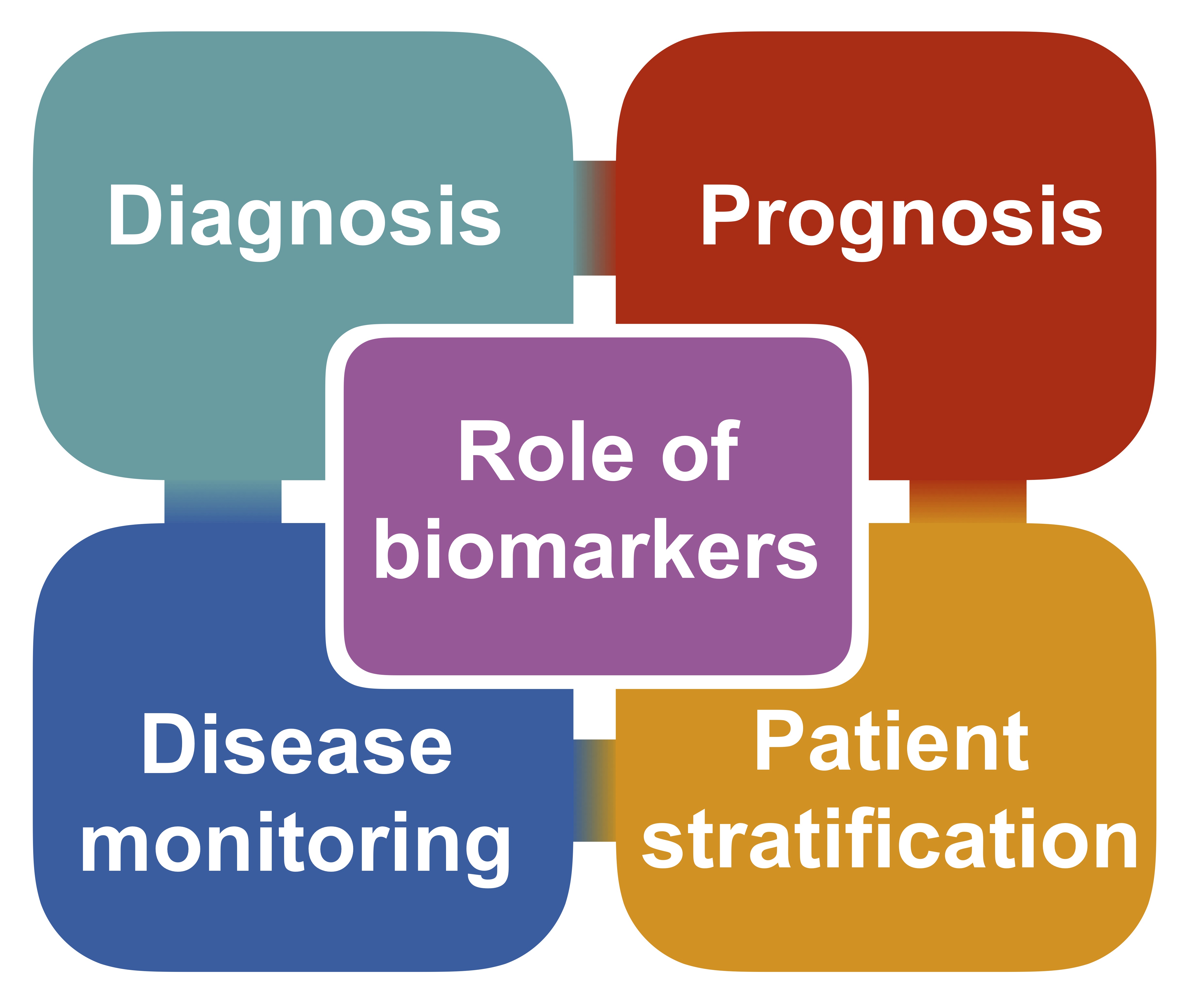
How do we approach the study of biomarkers?
The focus of our research is on the development and validation of novel biomarkers of motor neuron disease. We approach this by investigating the electrical properties of nerves and muscles at different stages of disease, often assessing patients at multiple timepoints throughout their disease. This provides the data required to understand the natural course of the disease process.
The following diagram depicts the two main biomarker trajectories over time. Some biomarkers are at their maximum before the disease begins (premorbid period), at which point they start to decline at a linear rate in the presymptomatic and symptomatic phases of the disease. A less steep decline implies less aggressive disease, which would be a favourable outcome for a novel therapeutic. A good example of this type of biomarker in MND is the motor unit number index, MUNIX (see section on motor unit number estimation).
Other biomarkers are at zero before the disease begins, at which point they rise rapidly and remain at a relatively constant level throughout the disease. Less aggressive disease is marked by a reduction in the level. A good example of this type of biomarker in MND is serum neurofilament light chain, which is detected in a blood test.
Motor neurons
Neurophysiology basics
What are motor neurons?
Motor neurons are split into two types. The upper motor neuron connects the brain to the spinal cord, and the lower motor neuron travels from the spinal cord to the muscle. The two types of motor neuron work in relay, whereby signals from the upper motor neuron are communicated to the lower motor neuron via a synapse in the spinal cord.
The destination of the lower motor neuron dictates the level at which this synapse occurs. For leg muscles, this is in the thoracic part of the spinal cord; but for tongue muscles, this is in the brainstem (before the spinal cord even starts).
Many motor neurons are therefore extremely long (e.g. over one metre). Consequently, it takes huge amounts of energy to ensure the correct proteins and molecules are in the correct part of the cell at the correct time. If any of this complex system goes awry, the motor neuron is vulnerable to deterioration.
What is a motor unit?
A motor unit comprises the lower motor neuron, its terminal branches and the muscle fibres it connects to. When activated, the lower motor neuron sends signals to the muscle fibres, telling them to contract.
The way these motor units adapt to the underlying disease process can be tracked over time.
Motor units share several key characteristics: (to update this line please)
Long distance transport
The axons of lower motor neurons cover vast distances for their size. From the spinal cord to the muscle is the equivalent of a tube train travelling from London to New York! Transporting the necessary proteins from the cell body in the spinal cord to the nerve terminal in the muscle is therefore an onerous and energy-consuming task.
Variation in size
If you were to cut a peripheral nerve in cross-section, neurons of all types (motor and sensory) would be seen tightly aligned. The wider ones are the lower motor neurons, able to transmit signals at the fastest speeds.
Terminal branching
As axons reach the muscle, they begin to branch off in order to connect to many muscle fibres simultaneously. These projections are long and plentiful, enabling a single motor unit to cover a large volume of muscle. This ensures the force generated by a single motor unit is sufficiently spread out.
Compensatory sprouting
Lower motor neurons have the capacity to create new branches to compensate for the loss of neighbouring motor neurons. This sprouting process has evolved as a protective mechanism, so that the connections with muscle fibres can be maintained. This helps to maintain muscle strength despite the loss of motor neurons.
Muscle weakness
Why does weakness develop?
It is estimated that a half of motor units can be lost before muscle weakness develops.
In motor neuron disease, this compensatory mechanism can only go on for so long. Once half of the original pool of motor units have died (due to the relentless nature of the disease), a tipping point is reached.
The sprouting process is no longer sufficient to maintain connections with all muscle fibres. Consequently, muscle fibres begin to fail leading to muscle wasting. It is the failure and loss of muscle fibres that leads to weakness.
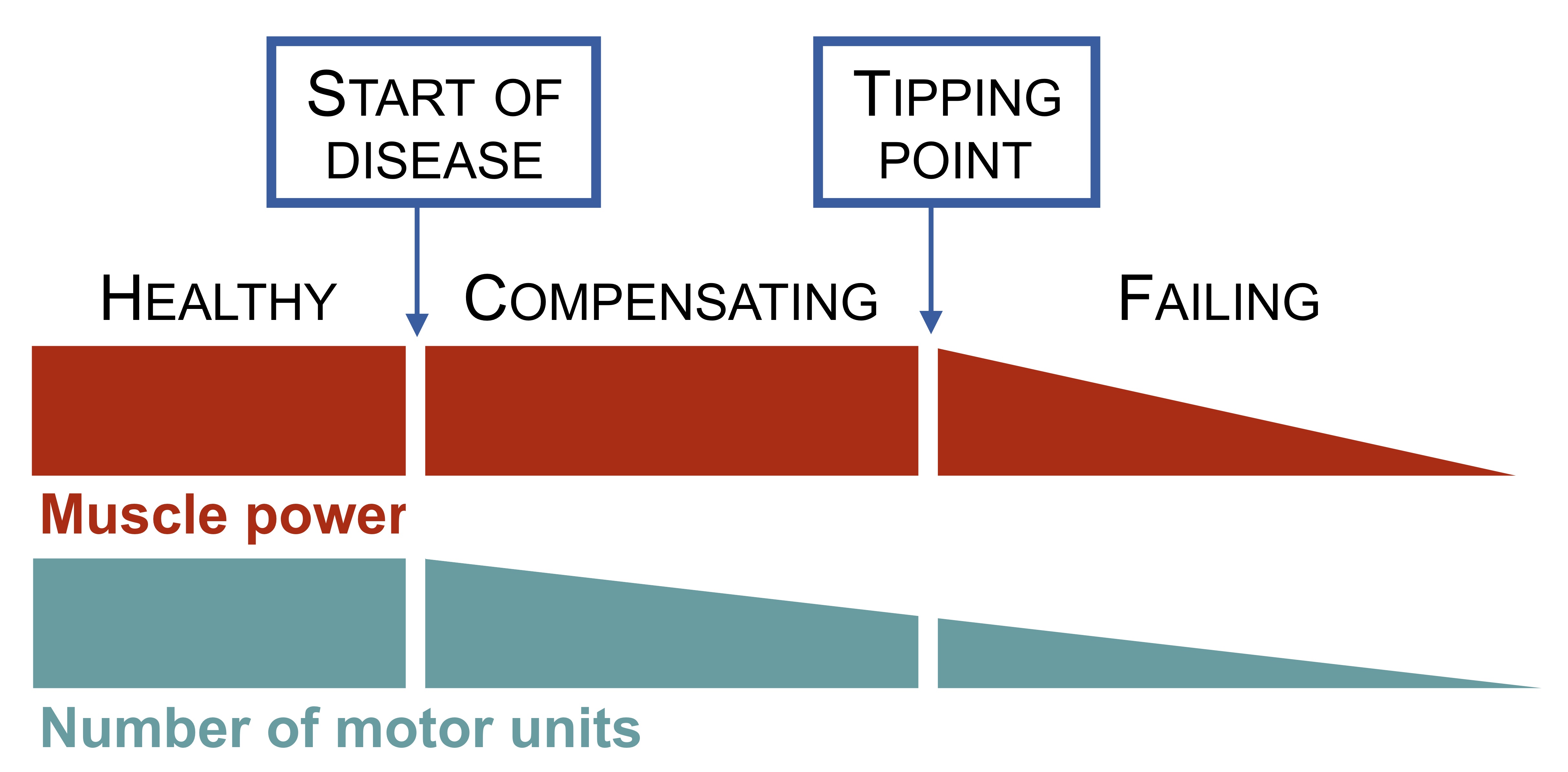
Fasciculations
What is a fasciculation?
A fasciculation is an involuntary muscle twitch, which arises from the spontaneous firing of a single motor unit. Usually, motor units are instructed by the brain when to become active and when to remain inactive. This precision is essential to ensure motor units fire simultaneously, thereby activating sufficient muscle fibres to generate a certain muscular force for a specific activity.
In some conditions, like motor neuron disease and benign fasciculation syndrome, motor neurons can become hyperexcitable, which can lead to random, solitary firing. Here is a summary of what we know about this process:
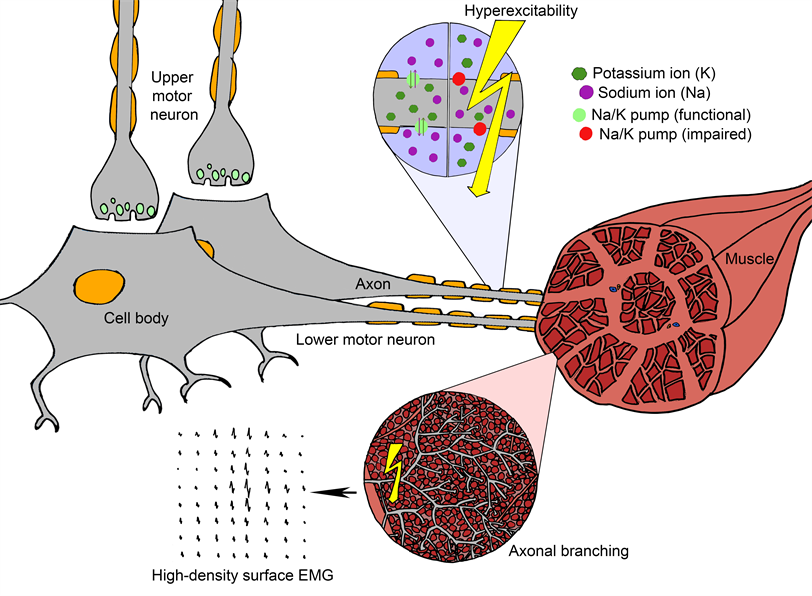
What causes fasciculation?
When the tightly regulated control of sodium and potassium ions across the neuronal membrane is impaired, the neuron can become hyperexcitable and is more likely to fire spontaneously. When it does, the electrical signal travels along the axonal branches deep within the muscle. This in turn leads to the activation of multiple muscle fibres, which we are able to detect with high-density surface EMG. Each motor unit produces its own ‘fingerprint’ so we can recognise when the same motor unit fires again and again.
Results from our research into fasciculations
We have applied the SPiQE algorithm to determine its clinical utility in MND, showing that a fasciculation frequency above 14/min in biceps brachii is a promising diagnostic aid in MND, while the rate of change of fasciculation frequency can forecast the aggressiveness of the disease. By tracking MND patients over the course of one year, we provided evidence of a ‘rise and fall’ pattern of fasciculation firing, which is best explained by a complex interplay between neuronal hyperexcitability, axonal loss and muscle fibre reinnervation. These data indicate the viability of fasciculation parameters as biomarkers of disease in MND.
See our Publications page for links to the full research papers.
What is high-density surface electromyography?
SPiQE is based on muscle recordings captured by high-density surface electromyography (or ‘HDSEMG’ for short). This relatively modern technique has many advantages over alternative, more traditional methods like needle electromyography.
- It's non-invasive and painless
- It can be repeated on multiple occasions for longer durations
- It provides enriched spatial information
Arranged in a square grid covering 40 square centimetres, 64 channels simultaneously record the underlying muscle activity. It’s like 64 eyes watching from slightly different angles, building up a comprehensive 3D picture of the electrical changes taking place. This is what makes HDSEMG so powerful.
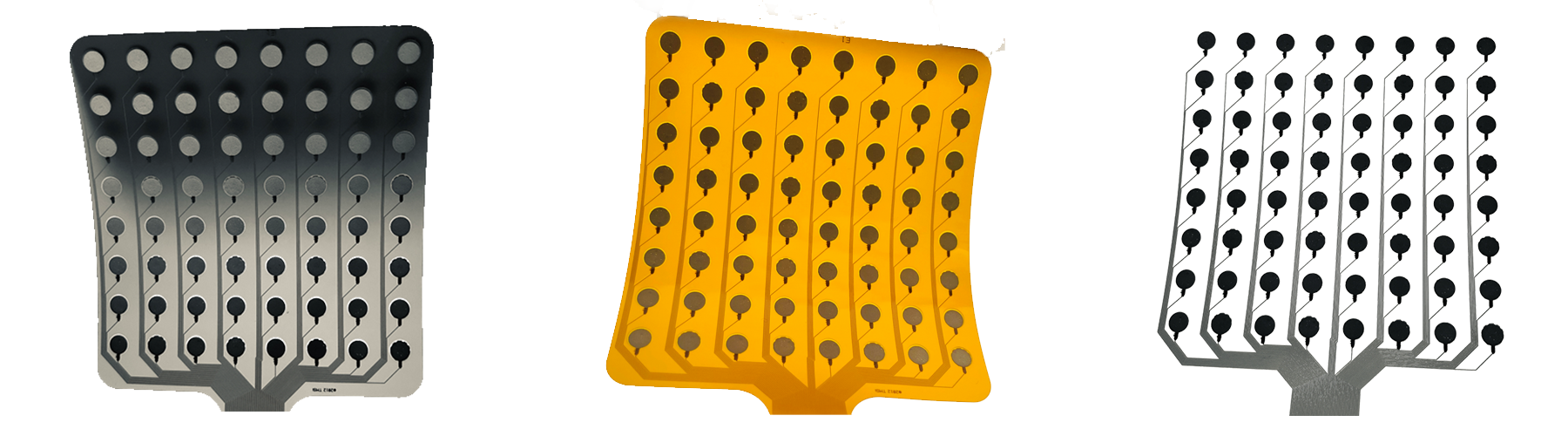
Take a look at this 2-minute video, which provides a basic explanation of SPiQE’s analytical process.